Pharma Supply Chains: Dramatic Changes Ahead
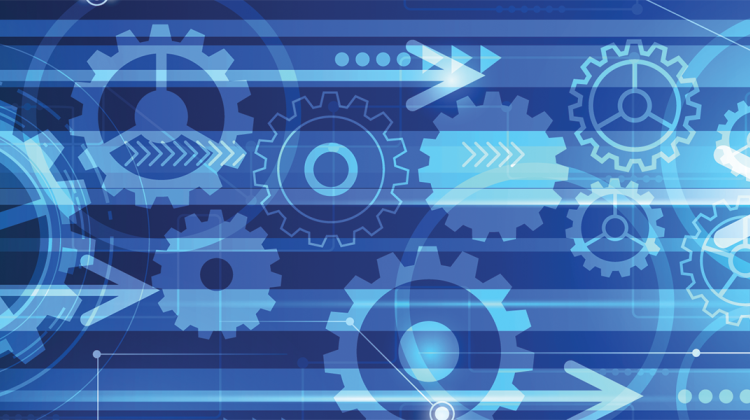
Biopharmaceutical supply chains are undergoing dramatic changes that will affect the design and operation of the facilities of the future. Predominant among these is the shift away from primary care to specialty products and personalized medicine, and from large-scale production of low-value small molecules to low-volume, high-value biologics.
This transition to personalized medicine bears no resemblance to the manufacturing platforms that exist today for monoclonal antibodies (mAbs) or vaccines. These are step changes in size, scale, or demand. In some cases, we might rely on the same manufacturing technology, but need 10 times as much of it. In others, we might need an entirely different platform.
Managing both the risks and costs associated with these significant changes requires nimble, creative thinking. And this, in turn, requires an influx of intelligent, skilled, and innovative employees. step rely no changes for resemblance on monoclonal the in same size, manufacturing technology, but need 10 times as much of it. In others, we might need an entirely different platform.
Facility Of The Future Or Dinosaur?
Since about 95% of current pharmaceutical revenues are derived from small-molecule products, ours is a small-molecule industry with biologics tacked on. About half of the research and development pipeline is devoted to biologics, however, and this could increase to 70%–80% by 2020.
Seeing this, some C-suite executives question the relevance of stainless steel plants, believing that those built in the 1980s and 1990s are outdated dinosaurs incapable of dealing with newer biologic products. Despite this, we continue to build plants similar to those built in the 1990s, in part because the bulk protein demand for biologics continues to increase, especially for those that expand into primary-care-sized markets. Since it takes six years to bring a large plant online, our current planning conversations extend to 2024–2026. For large-scale stainless steel, 2023 is tomorrow.
As an industry, we will have invested an additional $10–$15 billion in stainless steel plants by the early 2020s, and the products we make in them will have 10–15-year life spans. At least three-quarters of the pipeline currently in Phase I or II consists of traditional mAbs that will not be licensed for three or four years. Much of this will land in large-scale plants; this technology will be with us for the better part of the next two decades.
Over the last 15 years, the pharmaceutical industry has undergone a rough transition. Patent expiries, a drop in R&D productivity, and reimbursement challenges have all put pressure on earnings. In response, we adopted supply chain efficiency initiatives from nonpharmaceutical industries, particularly automotive manufacturing. Manufacturing costs are a larger portion of the costs of goods in those industries, so they were early leaders in cost-reduction and supply chain efficiency.
One way that we made pharmaceutical supply chains more cost-effective was to increase utilization. Automotive and chemical manufacturing, for example, must run at a utilization approaching 95% to be profitable. Twenty years ago, in contrast, it was common for pharmaceutical companies to operate at 55% utilization. That number has since increased to 85%.
One supply chain change in API production has been the shift from internal manufacturing to outsourcing. Because these products can have many synthesis steps, it’s good to have redundancy in sourcing—but that can be hard to see. A supply chain for a product may look dual-sourced, but if a single step in those dual routes relies on a single producer, you have a sole-source supply chain. Issues like these have the potential to increase product supply and quality problems.
Externalized API production can also be subject to local market stresses. Fifteen years ago, much of our production was in Europe and North America. Now, companies have significant portions of their drug substance supply—in some cases more than 60%—in emerging markets. If API production is no longer internal, we can’t just assume that the contract manufacturing organizations (CMOs) on which we depend have the same level of commitment to quality metrics and standards that we do.
It’s possible to get there, though, and it has been done. Success depends on the companies that supply us having the desire to share our quality values. If CMOs combine commitment to a quality culture with rigorous analytics and quality metrics, then success in product supply can be more narrowly defined as product knowledge transfer.
A country’s culture can also affect a company’s ability to respond. A good example of this occurred when the Iron Curtain fell and Eastern Europe became an accessible market. At that time, Poland’s manufacturing plant standards were primitive by Western standards, but the desire to become best in class was there. All we had to do was show them the standards and get out of their way. Polish plants have since had a history of world-class production.
In other emerging markets, quality wasn’t as prominent an issue; securing the greatest profit from a plant was. In those cases, we found that cultural mindsets can be di cult to change. But even in some of those locations, we’ve seen progress. In China, for example, the government has made it quite clear that quality matters in pharmaceuticals. Policies are enforced. As a result, we achieve the same level of quality in our AstraZeneca China plants as we do anywhere else in the world.
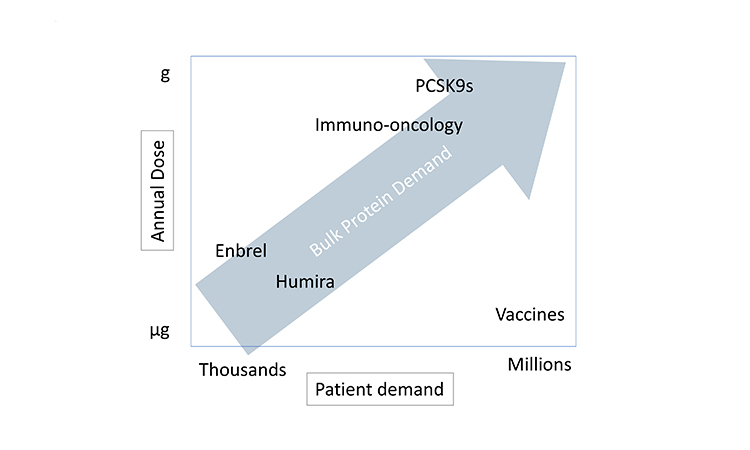
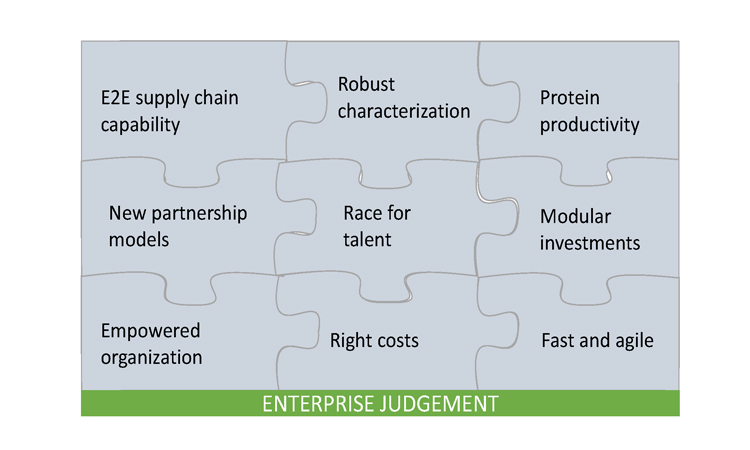
Industry Shifts
One driver of the dramatic change we’re seeing is the shift from predominantly primary care to specialty products. What does this imply?
- Primary-care drugs usually have multiple assets in class; specialty-care drugs are often sole assets in class.
- Specialty-care drug shortages can have an immediate and significant effect on patient health.
- Primary-care products are relatively high volume, lower value. Specialty-care products are relatively low volume, higher value. The distribution network size and risk profile are different.
- Primary-care products are more commonly mature products; their supply chain focuses on the balance of cost vs. resilience.
- Specialty-care products are newer, with unpredictable demand curves. These supply chains focus on resiliency and agility, especially in launch and early market years.
Statins are a good example of a primary-care product. There are half a dozen major brand-name products and multiple generic versions. If one company were to have a problem producing its statin, a physician could write a prescription for another with little or no effect on patient health. Risk management in this market can—and should be—different from risk management for a specialty-care market.
Specialty-care products target small, narrowly defined patient populations with severe, often life-threatening diseases. Approved products are often sole asset in class—meaning no other treatment option is available—and their complex risk/benefit profiles require deep scientific, clinical, and business expertise. Effects on patient health in the event of a supply shortage are often unavoidable, immediate, and potentially severe.
A specialty product supply chain must be managed differently. AstraZeneca’s Synagis,∗ for example, has a much smaller patient population than a typical primary-care product and is a sole asset in its class. It’s also a seasonal product that must be available when needed. If that market is shorted, you can calculate the potential morbidity and mortality with almost mathematical certainty. That is not the kind of math that I personally ever want to have to do.
As a result, these types of products must also be managed with a different risk model than typical primary-care products. We operate this supply chain with as close to minimal risk as possible. We maintain a minimum of one season’s worth of inventory, and dual-source production at every step, both internally and with a CMO that we’ve relied on for over 15 years. This same risk profile can apply to immuno-oncology drugs, which are sole assets in class for certain indications that have patient survival profiles measured in months. If one of these drugs experiences a supply shortage, there is no time to rebuild inventory before the shortage affects patient health.
The supply chain for a new product introduction (NPI) must be more agile and flexible than that of a mature product, since potential demand is uncertain and approval times can accelerate dramatically. NPIs are more di cult to outsource because of these uncertainties. The greatest risk they present to industry is the potential inability to supply enough drug quickly. The result of not supplying sufficient product upon launch could be devastating and would almost certainly affect patient health. These problems will be exacerbated as biologics move into primary-care-sized markets for certain indications (e.g., first-line oncology, Alzheimer’s disease).
Marketplace Disruption
We now have the tools in hand to make the transition to specialty products. The issue is to understand how to manage the supply chain for novel biologic products in general and specialty-care products specifically, and recognize the differences between them. That is disruptive, because companies have structured their supply chains to work very efficiently in one traditional marketplace. Now they have to be good in two very different marketplaces.
It will be disruptive because after spending the past 15 years outsourcing almost all API production we now must decide whether that is appropriate for those portions of our network focused upon NPIs. Our executive committees and boards, which have many other demands for investment, including pipeline development, will have to analyze the costs associated with these changes.
* A vaccine used to prevent respiratory syncytial virus infections, especially in premature infants
Option 1 | Option 2 | Option 3 | Option 4 | Option 5 | Option 6 | |
---|---|---|---|---|---|---|
Batch | Batch with continuous | N-1 with batch | N-1 with continuous | Perfusion with batch | Perfusion with continuous | |
Upstream mode | Batch | Batch | N-1 perfusion | N-1 perfusion | Continuous | Continuous |
Downstream mode | Batch | Continuous | Batch | Continuous | Batch | Continuous |
Base titer (g/L) vvd | 8 | 8 | 10 | 10 | 0.95/1.5 | 0.95/1.5 |
Scale | 12.5 kL SS | 12.5 kL SS | 12.5 kL SS | 12.5 kL SS | 2 kL SUB | 2 kL SUB |
It will be disruptive for firms that are being pressured to manage inventory. Having an additional $1 billion tied up in inventory is the same, in economic terms, as building a new plant for that amount. I can envision models that could double a company’s current inventory, which represents a huge outflow of cash. More than $13 billion is currently dedicated to new biotechnology facilities, in planning or in flight, that will be required to support NPI production. Companies are also plowing a tremendous amount of cash back into R&D.
The transition will be disruptive if we decide that supply chain risks mean that certain parts of our network can’t be run at 85% utilization and need to run at 60% instead. In certain areas of our network this could take us back to the utilization rates of 15 years ago.
Speed to market will feel disruptive. We’re building plants for products that are only in Phase I trials. We’re accustomed to placing a purchase order for an API we’ll need in 18–24 months. Now we need five to six years to plan for a product that doesn’t even have an assigned product number.
Decisions, Decisions
It is common for CMOs to achieve mammalian cell culture titers in the range of 2.5–4.5 grams per liter (g/L). We can achieve titers of 6–8 g/L, and some R&D development products can reach 10 g/L. AstraZeneca developed small-scale biologics drug substance capacity specifically designed for high-titer, low-volume production where it’s appropriate. This eliminates the need to run our large-scale facilities for inefficient short runs of multiple products.
Must a facility of the future mean bricks and stainless steel? Or can it mean doubling the throughput of assets you already have? The maxim “sweat the asset,” drawn from other industries, is one we take seriously. What does this mean in real life?
Our Frederick, Maryland, facility is a typical 4 × 15,000-L large-scale mAb plant. In two years it has improved titers, cut cycle times, and reduced turnaround times to improve operating yield by 80%. The network essentially added a new plant without having to build one. And if you want a measure of what that achievement is worth in capital savings, a “Frederick”-type plant would cost on the order of $800 million to build and license.
What does that mean for planning new pipeline products? Let’s assume, without getting into specifics, a key product demand of 3,500 kilograms at the bioreactor stage. At a titer of 2.5 g/L, a cycle time of 5.5 days, and a turnaround time of 3.0 days, that requires 168 lots or 3.0 “Fredericks.” At a titer of 7.0 g/L, a cycle time of 3.75 days and a turnaround time of 1.0 days, that requires 60 lots, or 0.7 “Fredericks.” By sweating your asset, you gain the equivalent of more than two new plants. To me, that is as much a “facility of the future” as new platform technology.
Accelerated regulatory approvals can be achieved in as little as three years, not the seven the industry has seen on average. We need to make decisions about a plant earlier than we traditionally would like to, but it still takes five to six years to build. As a result, we must anticipate volumes as well as the type of process platform that will be needed years ahead of any certainty regarding product approvals and demand.
We ask the R&D team to land new products on those existing platforms. As an example, we have limited perfusion capacity in our internal network. We ask that development avoid perfusion if possible, but there will be products for which there is no other option. Then we will have to respond, either via outsourced capacity or by modifying internal plants.
Another decision involves the adoption of single-use technology (SUT), whose key operating and capital cost advantage is that it replaces fixed stainless bioreactors (and other vessels) with disposable plastic-bag units that can be discarded post-use. We use SUT throughout our network, but not at the large-scale bioreactor stage. It is people-intensive, has different standard operating procedures, and may not be useful for all products. It is not simply interchangeable with fixed stainless steel. It is also available only up to 3,500-L scale, which narrows its potential use.
Deciding whether to use batch processing or continuous manufacturing (CM), another disruptive technology, depends on the type of drug being manufactured. In the small-molecule space, CM is the way to gain efficiency. For biologics, the situation is more challenging, because there is no definitive test that can determine final drug product efficacy. Guaranteeing that the manufacturing process is within the ranges and specifications used to make that product for clinical trials is currently our only measure of product efficacy. In other words, “the process is the product.”
When a process deviation occurs, ring fencing those lots of product that might be affected is of critical interest. In a typical biologics batch process, we are dealing with multiple discrete lots, and can usually set the ring fence boundaries to limit the lots at risk—often to one lot. With continuous processing (oil refining is a classic example), what is the production boundary of the affected material? The ring fence? One day? One week? One month? We will solve this problem, but the challenges of converting to a continuous process are not limited to developing the manufacturing technology itself.
Control Networks
For blood fractionation, the control network is designed to keep blood sourced from patients with certain diseases out of the supply chain. Once we are certain that none of the blood or plasma came from a problematic source, then that pooled batch no longer needs to be tracked.
Personalized medicine, however, would benefit from having all parts of its supply chain under one roof—from the first extraction from the patient, to the lab where the drug is made, through the cold chain, and then to reinjecting the finished product into the same patient. In practice, this will be very di cult to do. For patient-specific products, managing this control network is the issue to be solved.
Personalized medicine facilities use many small bioreactors, each producing a separate patient-specific product. From a supply chain and a regulatory affairs perspective, this complexity, while necessary, is daunting. Everything that touches the product, every bioreactor it goes into, every test it undergoes in the process must be tagged with the patient’s name and controlled to ensure that the final product goes back to the patient from whom the original serum was drawn. If we can’t demonstrate, without fail, that nothing adverse happened anywhere in that chain, then the product will be rejected and cannot be administered. These patient-specific supply chains will also be very time sensitive.
Managing Risk And Cost
As we embrace the facility of the future, we must understand that we’re managing risk—and it can be di cult to perceive hidden or consequential risk. We are not the automotive industry; our risk profile and tolerance are a lot different from General Motors’s or Toyota’s. It’s surely a lot different from someone who manufactures sneakers.
Keeping track of interconnecting nodes in the supply chain is like playing nine-dimensional chess. We must imagine moving a piece to see how it will affect the movement of product many steps later. We must evaluate scenarios in which something unforeseen could affect other parts of the supply chain, then answer three questions:
- What is the risk that a failure will occur?
- What is the impact, the severity of the failure?
- Is the patient outcome acceptable?
If a hypothetical scenario has only a 1% risk of failure but the result would be catastrophic, then that risk must be examined in great detail. At the same time, we can’t allow ourselves to be paralyzed by risk because we must ultimately produce the product.
Diverse Skills Needed
The thing I love about biologics is that technology touches every aspect of production. Regardless of your level of management, you are never out of touch with that technology. It is a mentally engaging business; solving these complex problems requires teams of all ages and levels of experience. We need people with broad intellectual curiosity, and we need plenty of them. With expansions in large-scale biologics drug substance facilities already underway, we estimate the industry will need as many as 30,000 highly skilled employees. More than two-thirds of these will have college degrees; 25%–35% of them will have advanced degrees and/or comparable years of experience.
These complexities will have a profound effect on the education of new employees. The learning curve isn’t limited to the technology, but includes the molecular biology of cell culture in a bioreactor and the engineering of new equipment. Consider enterprise-wide cold chain systemic control, from plant to patient. This is a level of supply chain detail that has never been done on a global scale. Diverse disciplines are involved, including mechanical engineering, software programming, automation, controls, chemical engineering, and biochemical engineering, to name a few.
The need to design and operate the facilities of the future is limitless. So are the opportunities for innovation and discovery.